Let us begin our explanation of how Newton changed our
understanding of the Universe by enumerating his Three Laws of Motion.
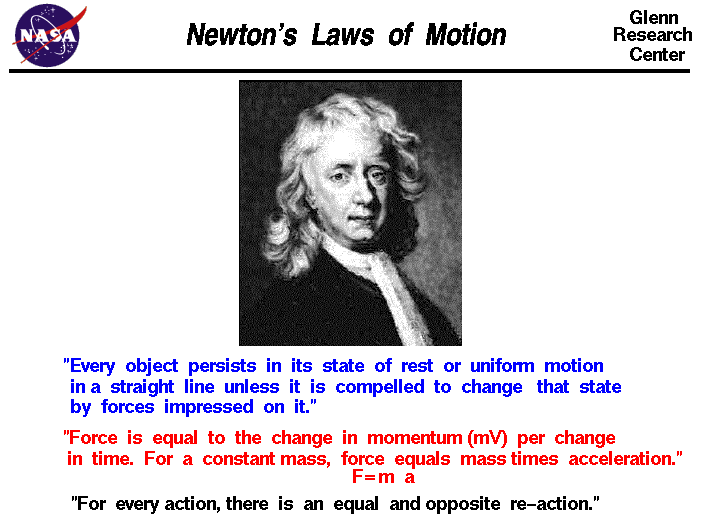
Newton's First Law of Motion:
Galileo defined inertia as the tendency of an object to maintain its initial state of motion. Newton related the concept of inertia to mass. Originally, Newton called mass a quantity of matter. But later redefined it as a measure of inertia. From this idea, it can be said that an object which is more massive has more inertia or has more resistance to change in motion than a less massive object does. For example, a truck has more inertia than a bicycle. Newton’s first law of motion, which is also known as the law of inertia, states that:
I. A body at rest remains at rest, and a body already in
motion remains in motion wit a constant velocity (constant speed and
direction), in the absent of an unbalanced applied force.
History of the Law of Inertia
Newton's first law is just a restatement of what Galileo had already described and Newton gave credit to Galileo. It differs from Aristotle's view that all objects have a natural place in the universe. Aristotle believed that heavy objects like rocks wanted to be at rest on the Earth and that light objects like smoke wanted to be at rest in the sky and the stars wanted to remain in the heavens. However, a key difference between Galileo's idea and Aristotle's is that Galileo realized that force acting on a body determines acceleration, not velocity. This insight leads to Newton's First Law—no force means no acceleration, and hence the body will maintain its velocity.
The law of inertia apparently occurred to several different natural philosophers and scientists independently. The inertia of motion was described in the 3rd century BC by the Chinese philosopher Mo Tzu, and in the 11th century by the Muslim scientist, Alhazen and Avicenna. The 17th century philosopher René Descartes also formulated the law, although he did not perform any experiments to confirm it.
ILLUSTRATION
The law of inertia is most commonly experienced when riding in cars and trucks. In fact, the tendency of moving objects to continue in motion is a common cause of a variety of transportation injuries - of both small and large magnitudes. Consider for instance the unfortunate collision of a car with a wall. Upon contact with the wall, an unbalanced force acts upon the car to abruptly decelerate it to rest. Any passengers in the car will also be decelerated to rest if they are strapped to the car by seat belts. Being strapped tightly to the car, the passengers share the same state of motion as the car. As the car accelerates, the passengers accelerate with it; as the car decelerates, the passengers decelerate with it; and as the car maintains a constant speed, the passengers maintain a constant speed as well.
But what would happen if the passengers were not wearing the seat belt? What motion would the passengers undergo if they failed to use their seat belts and the car were brought to a sudden and abrupt halt by a collision with a wall? Were this scenario to occur, the passengers would no longer share the same state of motion as the car. The use of the seat belt assures that the forces necessary for accelerated and decelerated motion exist. Yet, if the seat belt is not used, the passengers are more likely to maintain its state of motion. The animation below depicts this scenario.
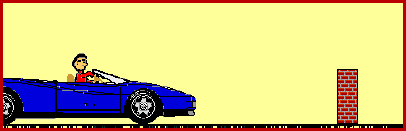
If the car were to abruptly stop and the seat belts were not being worn, then the passengers in motion would continue in motion. Assuming a negligible amount of friction between the passengers and the seats, the passengers would likely be propelled from the car and be hurled into the air. Once they leave the car, the passengers becomes projectiles and continue in projectile-like motion' Now perhaps you will be convince of the need to wear your seat belt. Remember it's the law - the law of inertia
Newton's Second Law of Motion:
II. The relationship between an object's mass m, its acceleration a, and the applied force F is F = ma. Acceleration and force are vectors (as indicated by their symbols being displayed in slant bold font); in this law the direction of the force vector is the same as the direction of the acceleration vector.
This is the most powerful of Newton's three Laws, because it allows quantitative calculations of dynamics: how do velocities change when forces are applied. Notice the fundamental difference between Newton's 2nd Law and the dynamics of Aristotle: according to Newton, a force causes only a change in velocity (an acceleration); it does not maintain the velocity as Aristotle held.
This is sometimes summarized by saying that under Newton, F = ma, but under Aristotle F = mv, where v is the velocity. Thus, according to Aristotle there is only a velocity if there is a force, but according to Newton an object with a certain velocity maintains that velocity unless a force acts on it to cause an acceleration (that is, a change in the velocity). As we have noted earlier in conjunction with the discussion of Galileo, Aristotle's view seems to be more in accord with common sense, but that is because of a failure to appreciate the role played by frictional forces. Once account is taken of all forces acting in a given situation it is the dynamics of Galileo and Newton, not of Aristotle, that are found to be in accord with the observations.
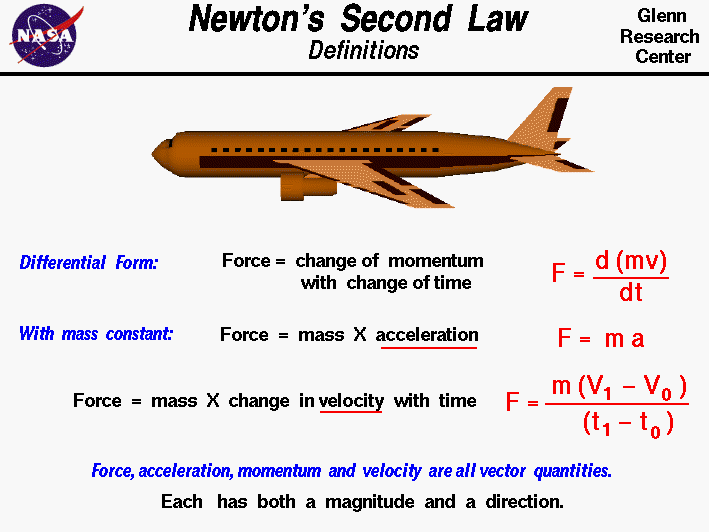
Sir Isaac Newton first presented his three laws of motion in the "Principia Mathematica Philosophiae Naturalis" in 1686. His second law defines a force to be equal to the differential change in momentum per unit time as described by the calculus of mathematics, which Newton also developed. The momentum is defined to be the mass of an object m times its velocity v. So the differential equation for force F is:
F = d(m * v) / dt
If the mass is a constant and using the definition of acceleration a as the change in velocity with time, the second law reduces to the more familiar product of a mass and an acceleration:
F = m * a
Since acceleration is a change in velocity with a change in time t, we can also write this equation in the third form shown on the slide:
F = m * (v1 - v0) / (t1 - t0)
The important fact is that a force will cause a change in velocity; and likewise, a change in velocity will generate a force. The equation works both ways. The velocity, force, acceleration, and momentum have both a magnitude and a direction associated with them. Scientists and mathematicians call this a vector quantity. The equations shown here are actually vector equations and can be applied in each of the component directions.
The motion of an aircraft resulting from aerodynamic forces and the aircraft weight and thrust can be computed by using the second law of motion.
Newton's Third Law of Motion:
III. For every action
there is an equal and opposite reaction.
This law
is exemplified by what happens if we step off a boat onto the bank of a
lake: as we move in the direction of the shore, the boat tends to move in
the opposite direction (leaving us facedown in the water, if we aren't
careful!
The statement means that in every interaction, there is a pair of forces acting on the two interacting objects. The size of the forces on the first object equals the size of the force on the second object. The direction of the force on the first object is opposite to the direction of the force on the second object. Forces always come in pairs - equal and opposite action-reaction force pairs. Consider the flying motion of birds. A bird flies by use of its wings. The wings of a bird push air downwards. Since forces result from mutual interactions, the air must also be pushing the bird upwards. The size of the force on the air equals the size of the force on the bird; the direction of the force on the air (downwards) is opposite the direction of the force on the bird (upwards). For every action, there is an equal (in size) and opposite (in direction) reaction. Action-reaction force pairs make it possible for birds to fly.
| ![]() |
To every action there is always opposed an equal reaction: or the mutual actions of two bodies upon each other are always equal, and directed to contrary parts. — Whatever draws or presses another is as much drawn or pressed by that other. If you press a stone with your finger, the finger is also pressed by the stone. If a horse draws a stone tied to a rope, the horse (if I may so say) will be equally drawn back towards the stone: for the distended rope, by the same endeavor to relax or unbend itself, will draw the horse as much towards the stone, as it does the stone towards the horse, and will obstruct the progress of the one as much as it advances that of the other.
If a body impinges upon another, and by its force changes the motion of the other, that body also (because of the equality of the mutual pressure) will undergo an equal change, in its own motion, toward the contrary part. The changes made by these actions are equal, not in the velocities but in the motions of the bodies; that is to say, if the bodies are not hindered by any other impediments. For, as the motions are equally changed, the changes of the velocities made toward contrary parts are reciprocally proportional to the bodies. This law takes place also in attractions, as will be proved in the next scholium.
The Third Law means that all forces are interactions, and thus that there is no such thing as a unidirectional force. If body A exerts a force on body B, simultaneously, body B exerts a force of the same magnitude body A, both forces acting along the same line. As shown in the diagram opposite, the skaters' forces on each other are equal in magnitude, but act in opposite directions. Although the forces are equal, the accelerations are not: the less massive skater will have a greater acceleration due to Newton's second law. It is important to note that the action and reaction act on different objects and do not cancel each other out. The two forces in Newton's third law are of the same type (e.g., if the road exerts a forward frictional force on an accelerating car's tires, then it is also a frictional force that Newton's third law predicts for the tires pushing backward on the road).
Sciencia,com 2009. All rights Reserved